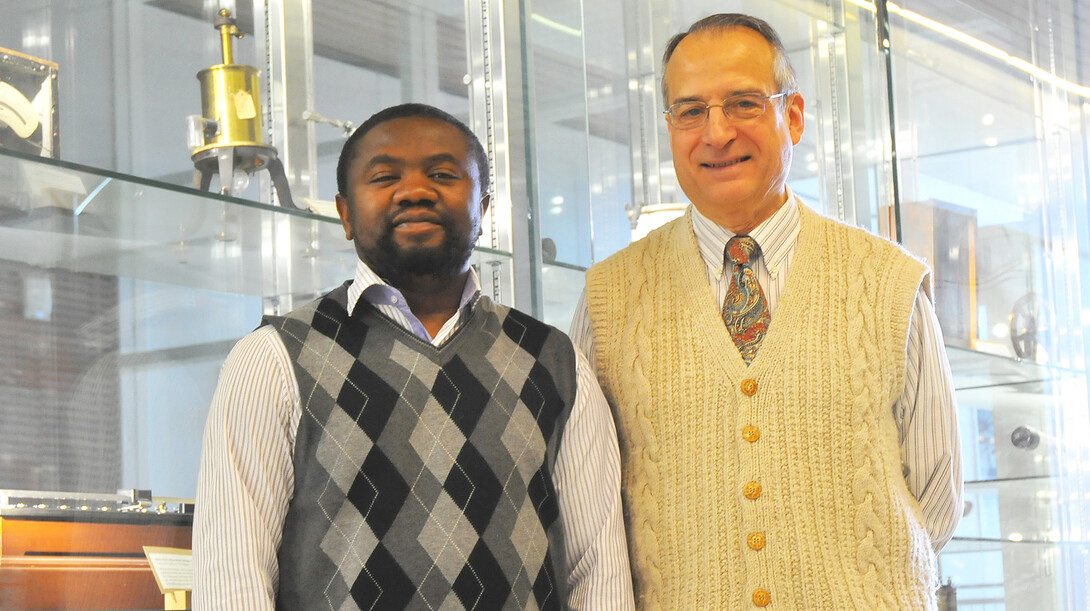
By solving a six-dimensional equation that had previously stymied researchers, UNL physicists have pinpointed the characteristics of a laser pulse that yields electron behavior they can predict and essentially control.
It’s long been known that laser pulses of sufficient intensity can cause ionization, producing enough energy to eject electrons from their ultrafast orbits around an atom.
An international team led by UNL researchers has demonstrated that the angles at which two electrons launch from a helium atom can depend on whether a laser pulse’s electric field is right- or left-handed — that is, whether it rotates clockwise or counterclockwise. The researchers have also calculated the distinct range of angles at which the electrons depart under both conditions.
The authors further confirmed that this effect, which they have coined “nonlinear dichroism,” appears only when an atom is struck by a sufficiently short, intense pulse featuring an elliptically shaped electric field.
The study, published in the journal Physical Review Letters, specifically determined that pulses capable of producing this effect last no longer than 200 attoseconds. Counting to one second by intervals of 200 attoseconds per second would take roughly 158.5 million years — longer than the span that’s passed since the end of Earth’s Jurassic period.
“The goal in laser atomic physics is to control electron motion and also image it,” said Anthony Starace, a George Holmes University Professor of physics who co-authored the study. “In order to do that, one needs probes that are much faster than the time scale on which electrons move.”
However, Starace noted that the exceptionally short duration of attosecond laser pulses — and the resulting quantum-scale interactions — can obscure the mechanics underlying laboratory outcomes.
“When things happen on such fast time scales, experimentalists don’t always know what they’ve achieved,” he said. “They cannot ‘see’ how electrons make atomic and molecular transitions. So they need means to ascertain, ‘How did we do that?’ or, ‘What did we have there?’”
The team’s paper, Starace said, should help laser physicists address this fundamental and prevalent issue.
“This has applications for timing electron processes,” Starace said. “On our human time scale, we think in terms of minutes, but these phenomena take place over inconceivably short fractions of a second. The question is: Are they 10 attoseconds? One hundred? A thousand? No one knows. With these results, we can explore theoretically — and fairly exactly — the question of how long electron transitions and processes take.”
By identifying and measuring nonlinear dichroism, Starace said, the team’s study also offers a new signature that quantum physicists can use to classify experimentally produced laser pulses and verify their results.
“If the experimentalists measure this new effect, they will have information as to how long their pulses are, what polarization their pulses have, and what the shape of their electric field is,” Starace said. “These are means to characterize their pulses.”
According to Starace, the study represents a significant step toward an even larger goal of laser physics: manipulating the most fundamental components of matter in the known universe.
“If experimentalists can finally produce such pulses reliably, this new effect allows great control over the way electrons move,” Starace said. “If we hit a target with the short attosecond pulses that have a particular duration and polarization, we can tell the electrons whether to go one way or another. This is humankind’s dream of controlling electrons, rather than just watching them.”
A numbers game
Jean Marcel Ngoko Djiokap, research assistant professor of physics, laid the path to the team’s findings by programming the first code capable of accounting both for the interactions between two laser-influenced electrons and the complexity of electric fields that move in multiple dimensions.
“Normally, theorists assume an electric field oscillates in one direction only, which reduces the scale of the computation,” Starace said. “With elliptical polarization, the electric field sweeps around in a plane. This adds another dimension to the problem, which greatly increases the numerical complexity and the difficulty of doing the calculation. Marcel has solved this problem.”
Starace likened the team’s computational approach to climbing aboard the elliptical “merry-go-round” of the laser pulse’s electric field. Performing calculations from this perspective — rather than the stationary perspective of those watching from outside — ultimately streamlined the problem, he said.
“If you’re on a merry-go-round, people on the sidelines see you spinning around – but to you, the horse you’re sitting on is stationary,” Starace said. “What Marcel has done is move the calculation from the laboratory frame [of reference] to this rotating frame, so that all we see is linearly polarized, one-dimensional light. The whole thing becomes simpler.”
Starace and Ngoko Djiokap co-authored the study with colleagues from Russia’s Voronezh State University; the Laboratory for Laser Energetics at the University of Rochester (New York); and Denmark’s Aarhus University.