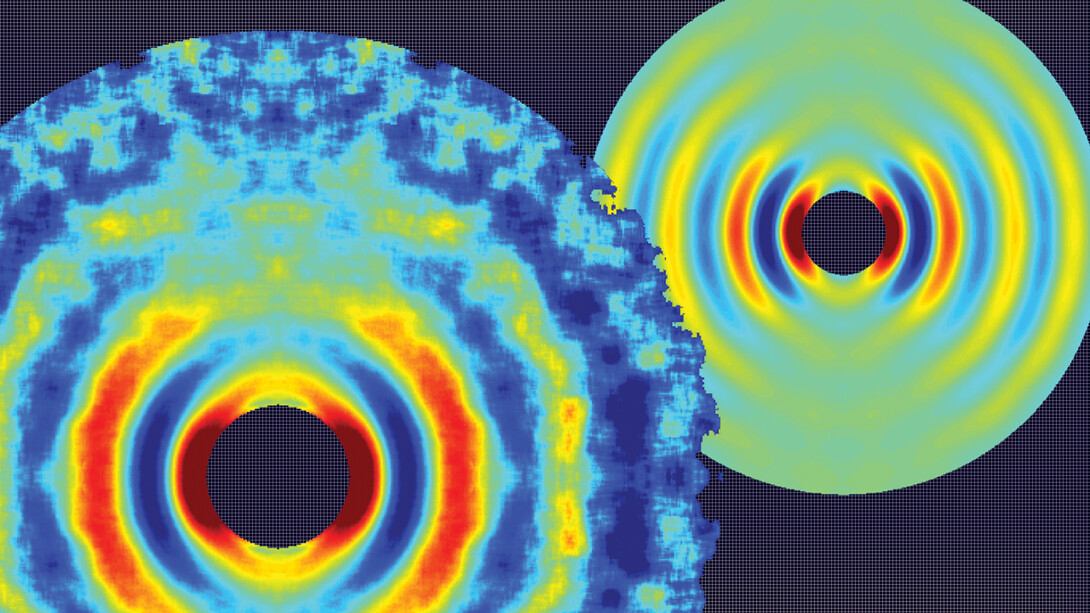
Physicists from the University of Nebraska-Lincoln, the SLAC National Accelerator Laboratory and Potsdam University are picking up good vibrations – via laser-driven excitations – after successfully recording the spring-like motion of a two-atom molecule.
As detailed in a study published Oct. 3 by the journal Physical Review Letters, the researchers captured the continuous motion of atomic nuclei in vibrating molecules for the first time. Recording this nuclear motion represents a milestone in the quest to produce “molecular movies” that could eventually unveil the dynamics of light-triggered chemical reactions.
“The main thing we want to see is when the molecular structure is changing,” said co-author Martin Centurion, associate professor of physics and astronomy at the University of Nebraska-Lincoln. “That we had not yet demonstrated (until now).”
Though molecules are always vibrating, light can excite their atoms into different structural configurations that result in photochemical reactions. While photosynthesis is considered the classic example, many other reactions regularly serve to help and harm – as when forming protective ozone in the Earth’s atmosphere, for instance, or the ultraviolet rays that cause skin cancer.
The researchers induced similar excitations by firing a laser at molecules consisting of two iodine atoms, which share a weak bond that alternately elongates and compresses like a spring when the atoms absorb light. That same laser, which resides at the Stanford University-housed SLAC lab, also triggered a short burst of electrons that raced toward the molecules at nearly the speed of light.
After measuring how the electrons diffracted from the laser-excited molecules – and comparing the patterns with those created when electrons struck undisturbed molecules – the researchers managed to calculate sequential positions of the molecules’ atoms. Those positions are essentially akin to frames that form a motion picture when stitched together, Centurion said.
Catching some waves
Similar to light, electrons exhibit properties of both particles and waves. The wavelike nature of light was famously demonstrated through the so-called double-slit experiment, in which a laser passing through two small openings produced not two points of light but instead an alternating pattern of bright and dark bands reminiscent of overlapping ripples in a pond.
That experiment also showed that the distance between slits dictated the interference pattern: closing the distance widened the dark gaps between the bands of light, whereas expanding that distance narrowed them. Because Centurion and his colleagues were firing electrons at many molecules oriented in various directions, their own interference patterns took the form of rings rather than straight bands. But just as in the classic double-slit experiment, the patterns of those rings changed in tandem with the distance between the iodine atoms.
That distance fluctuated by about 50 percent, which Centurion described as a “pretty big” amount. Yet the time it takes is anything but: An iodine molecule cycles through one springing motion in about 400 femtoseconds, which compares to one second as one second compares to roughly 80,000 years.
The researchers chose to work with a two-iodine molecule partly because physicists are familiar with its dynamics, making it a fitting test subject.
“We knew exactly how we should hit it to get this vibration,” Centurion said. “In a sense, we’re still trying to take a known system and test our apparatus with that system.”
The great unknown
Centurion said he and his colleagues are also setting their sights on less familiar, and more complex, molecular reactions. Those efforts will include trying to capture how nuclei respond when lasers of sufficient energy actually break atomic bonds, rather than simply distort them.
“Basically, we want to now go to a case where what happens is unknown,” he said. “We now have an instrument with which we hope to be doing some new science.”
The staggering complexity of photochemical reactions in molecules featuring even a few atoms, Centurion said, makes the prospect of capturing their nuclear movements an especially appealing prospect.
“If we can see these things as they’re happening, then there can start to be more feedback between theory and experiment,” he said. “They can feed on each other and hopefully develop something that’s good enough to be somewhat predictive.
“And if you can really understand these reactions and predict them, then you can maybe design (a molecule) that has certain properties. You could attach it to a (therapeutic drug) or use it to help transfer solar energy from a panel to a battery. I think we’re still very far from that, but that’s the goal.”
The study was authored by 20 researchers, including Centurion; Jie Yang, a former doctoral advisee of Centurion’s now at the SLAC lab; Markus Guehr, staff scientist at Stanford’s PULSE Institute; and Xijie Wang, research technical manager at the SLAC lab. Physical Review Letters has designated the study an “Editor’s Suggestion.”
The researchers’ work was funded in part by the U.S. Department of Energy’s Office of Science.
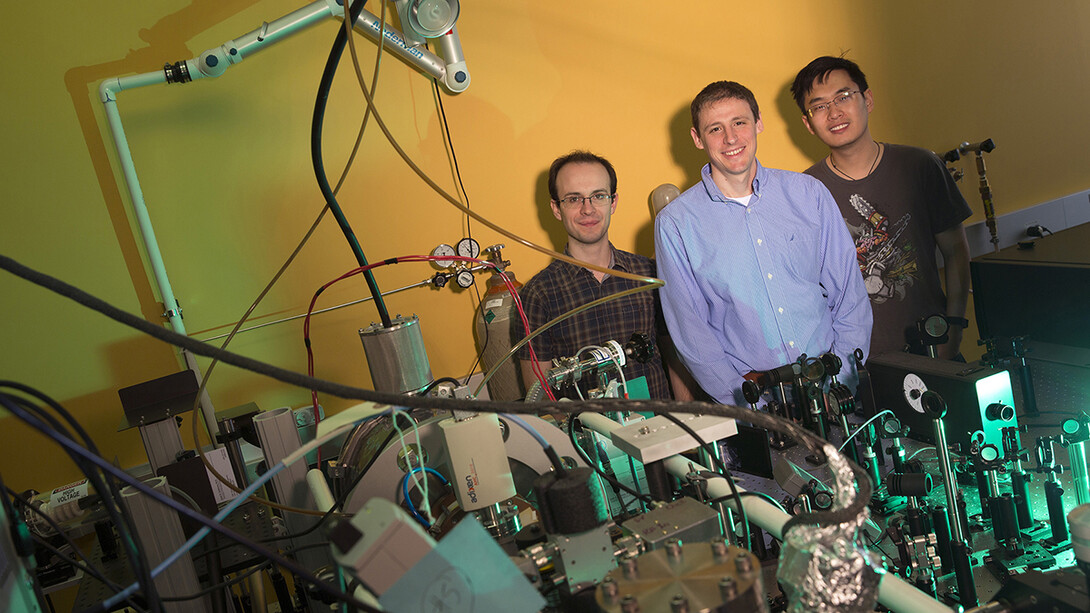