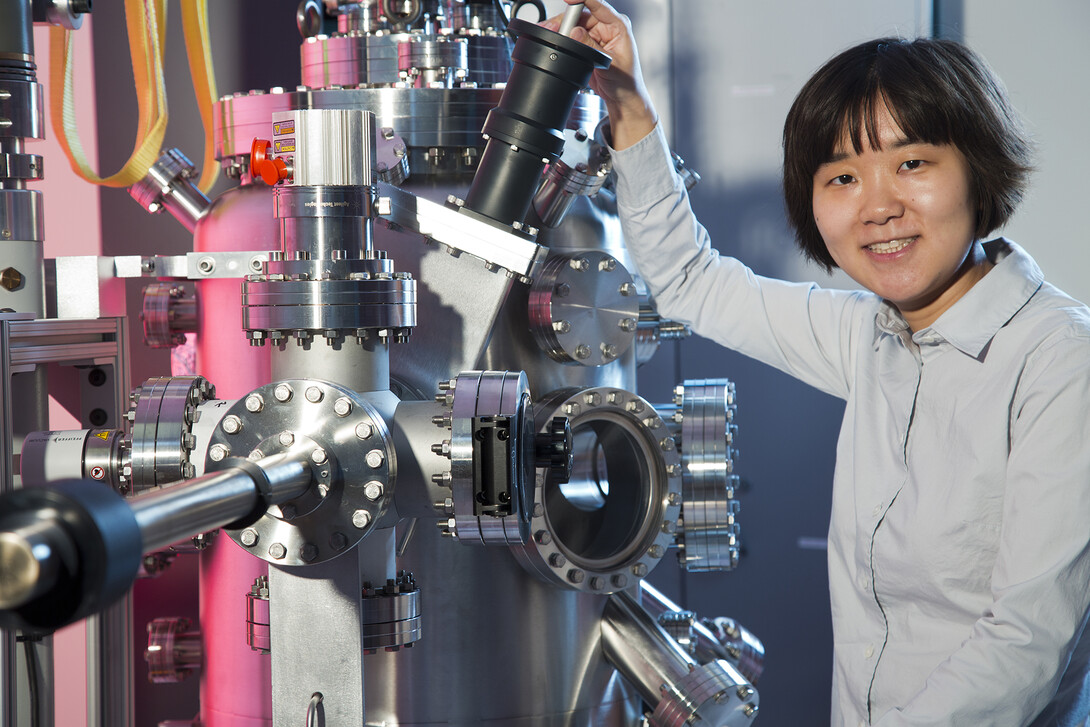
A team of Husker physicists and engineers has published a discovery that could help a promising material overcome a long-standing barrier to its implementation in digital storage and processing.
What kind of material is it?
It’s called lanthanum strontium manganite, and it features multiple properties that make it an appealing candidate for digital technologies. Chief among those properties: Its resistance to electric current can quickly change, by enormous amounts, when it’s subjected to a magnetic field.
The manganite holds particular promise for use in spintronics, an emerging class of technology that relies on the alignment of electrons’ spin – a measure of their angular momentum and magnetism – to encode data in the binary language of 1s and 0s. (When an electron’s spin points in one direction, it’s read as a 1; when it points the other way, it becomes a 0.)
So what’s the problem?
For all of its useful qualities, the manganite typically features a low level of anisotropy – a property that makes a material more prone to magnetize in one direction than another. When anisotropy is low, it’s easier to switch between one spin orientation and the other, which actually facilitates the writing of binary data.
But too little anisotropy limits the ability to control that spin in the first place, said Xia Hong, assistant professor of physics and astronomy. That fact, in turn, diminishes the stability of stored data.
“If you don’t have enough anisotropy, the spins are free to rotate in whatever direction,” Hong said. “So there’s effectively no (data) retention.”
And the solution was…?
The researchers first laid down a six-nanometer-thick film of the lanthanum strontium manganite on a template material called strontium titanate. (The film is approximately 15,000 times thinner than a human hair.) Hong’s team then patterned the top two nanometers into stripes that were 100 to 200 nanometers wide and separated by gaps of the same width.
The result? A 50-fold increase in anisotropy that approaches the value of cobalt, a material currently used to fabricate thin films in many hard drives.
According to Hong, the stripes induced structural changes in the atomic lattices that form the manganite. Though those atoms are normally equidistant from one another, the nanostripe pattern helped smush some of the top-layered atoms closer together. This disruption of the material’s equilibrium ultimately contributed to the leap in anisotropy.
“It’s a very surprising result,” Hong said.
Why is that?
Partly because it was accidental. Hong and her colleagues were actually trying, unsuccessfully, to lower the magnetic threshold at which the manganite’s large electrical response would kick in.
“My student was very disappointed,” Hong said. “I said, ‘OK, since we already have this nice material, why don’t we just measure its other (properties)?’
“This is the fun part of doing science, I think. There are all sorts of new discoveries waiting for you.”
Where can I learn more?
In the journal Physical Review Letters, which published the team’s study. Hong authored the study with Nebraska doctoral students Anil Rajapitamahuni and Le Zhang; Mark Koten, postdoctoral researcher in mechanical and materials engineering; John Burton, research assistant professor of physics and astronomy; Evgeny Tsymbal, George Holmes University Professor of physics and astronomy; Jeffrey Shield, Robert W. Brightfelt Professor of mechanical and materials engineering; and Vijay Raj Singh, now a postdoctoral researcher at Boston University.
The Nebraska Materials Research Science and Engineering Center, one of 23 such U.S. centers funded by the National Science Foundation, supported the team’s research. That research was conducted, in part, at the Nebraska Center for Materials and Nanoscience.