
Before defending, let alone counterattacking, comes reconnaissance.
It’s as true in the realm of infectious disease as combat. And the University of Nebraska–Lincoln’s Limei Zhang subscribes to the maxim that best encapsulates it.
“In order to fight your enemies, you have to know how your enemies survive and attack you,” said Zhang, associate professor of biochemistry. “That’s the fundamental question we are looking at.”
The enemy, in this case, is Mycobacterium tuberculosis. It is, in fact, a bacterium, and it does, indeed, cause tuberculosis, which kills about 1.5 million people annually — more than any other infectious disease. Nearly one-quarter of the global population is infected with the airborne bacterium. Though M. tuberculosis can lie dormant in people for years, an estimated 10% of those latent infections eventually turn active, at which point M. tuberculosis typically strikes the lungs. A chronic cough, bloody mucus, fever and weight loss frequently follow.
Several antibiotics can treat M. tuberculosis infections. Some strains of the bacterium, though, have evolved resistance to the two antibiotics typically used against them, with other strains now displaying resistance to secondary antibiotic options, too. The difficulty of discovering or engineering new antibiotics is spurring researchers like Zhang to seek overlooked, understudied components of infectious bacteria that might emerge as feasible targets for new drugs.
“Mycobacterium tuberculosis is a good example of how urgently we need to be looking for new approaches or identifying new targets,” Zhang said. “At the beginning, we had limited sources to fight against tuberculosis. Now, with it gaining antibiotic resistance, it’s even harder for us. We have to plan ahead and prepare.”
One of those targets, and the focus of a recent study from Zhang’s lab, is WhiB7: a unique type of so-called transcription factor that helps control the production of cellular machines, or proteins, whose instruction manuals are encoded in DNA. Churned out by M. tuberculosis, WhiB7 helps confer resistance against multiple antibiotics, getting called into action when the bacterium senses their presence.
As a transcription factor, WhiB7 has been shown to activate 50-plus genes residing within the bacterium’s DNA. One of those genes directs the construction of proteins that effectively pump antibiotics out of cells, sparing M. tuberculosis from their wrath. Another modifies how the bacterium builds its proteins, thwarting antibiotic efforts to halt their production.
Zhang’s team recently managed to characterize WhiB7-housed components down to the atomic level, capturing snapshots of a biochemical process that keys the bacterium’s campaign against antibiotics. That’s an especially critical milestone when it comes to understanding proteins, whose structures — particularly the way they fold into origami-esque arrangements — dictate their function and offer valuable hints about how they operate. Yet it’s just the second protein in the WhiB family to be profiled in such detail, joining WhiB1, which M. tuberculosis needs to survive even under normal conditions.
“In order to understand how it works, you have to know the shape,” Zhang said. “That’s the starting point for understanding its mechanisms.”
Both WhiB7 and WhiB1 contribute to the welfare of M. tuberculosis by sensing and coping with environmental cues. They begin by binding to a more general transcription factor, the primary sigma factor, which itself binds to a certain region of gene-adjacent DNA for the sake of calling over an enzyme called RNA polymerase. That RNA polymerase transcribes and copies the instructional code of the target gene into a strand of messenger RNA, which then facilitates the building of other proteins that carry out various tasks — among them, combating antibiotics sent in to destroy the bacteria.

Zhang and her colleagues uncovered multiple similarities between WhiB7 and WhiB1, down to the fact that they bind to the same portion of the primary sigma factor. Both proteins likewise rely on clusters of iron and sulfur atoms to make that binding work. (Those iron-sulfur clusters, which emerged before the first life on the planet, also help M. tuberculosis sense the onset of oxygen, nitric oxide and other endangering molecules that signal antibiotics are near.)
But the team discovered some major structural differences, too. It investigated the significance of those differences by transplanting the unique structural signatures of one protein to the other, creating chimeras that were mostly WhiB1 but partly WhiB7, or vice versa. If the behavior of WhiB1 changed after swapping in a single component from WhiB7, for instance, the team could be relatively confident that the component was responsible for the change — and functions similarly in the native WhiB7 protein.
One of those unique WhiB7 components, which resembles a fish hook, appears to strengthen the crucial binding between the transcription factor and DNA. The hook also seems to kick-start a reconfiguration of the DNA that enables its transcription into messenger RNA, making the hook a literal and figurative lynchpin in producing anti-antibiotic proteins.
Another difference? WhiB7 lacks certain molecular configurations that grant WhiB1 much of its structural stability, meaning that WhiB7 is less resilient to oxygen and other reactive molecules it encounters when under threat from antibiotics. The revelation initially surprised Zhang and her team: Reactive molecules can especially destabilize iron-sulfur clusters, potentially weakening the binding between WhiB7 and the primary sigma factor that is so essential to fighting antibiotics.
But the team’s findings suggest that the binding of WhiB7 to RNA polymerase helps protect the iron-sulfur clusters by reducing their sensitivity to oxygen, compensating for WhiB7’s inherent instability. And that instability may otherwise serve M. tuberculosis, Zhang said, by allowing WhiB7 to be dismantled and removed from a cell when antibiotics are not around, preserving energy for more pressing tasks.
Zhang said there’s much more reconnaissance to be done on WhiB7 and other bacterial proteins that help marshal resistance to antibiotics. Any of the structural and functional specifics her team has recently uncovered might ultimately prove vital in the quest to develop a new antibiotic for which existing M. tuberculosis strains have no answer. Which ones? It’s unclear, for now.
Still, Zhang has begun collaborating with Nebraska’s James Checco, assistant professor chemistry, to discuss how the latest scouting report might eventually be brought to bear on the ongoing, high-stakes battle. The duo is exploring the design of a peptide that can bind with members of the WhiB family and, consequently, might prevent those WhiB members from instead binding with transcription factors.
“Could we design a drug or intercept any part of this process by targeting WhiB7 to attenuate or kill Mycobacterium tuberculosis?” Zhang said. “That’s the ultimate goal.”
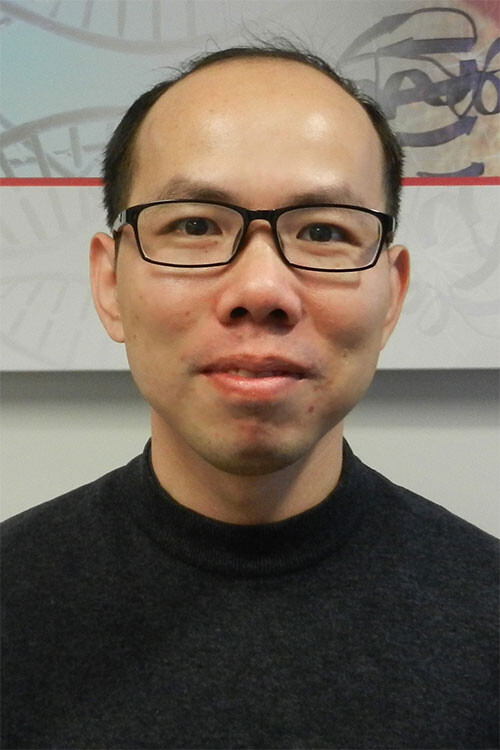
The researchers received support from the National Institutes of Health and the National Science Foundation.