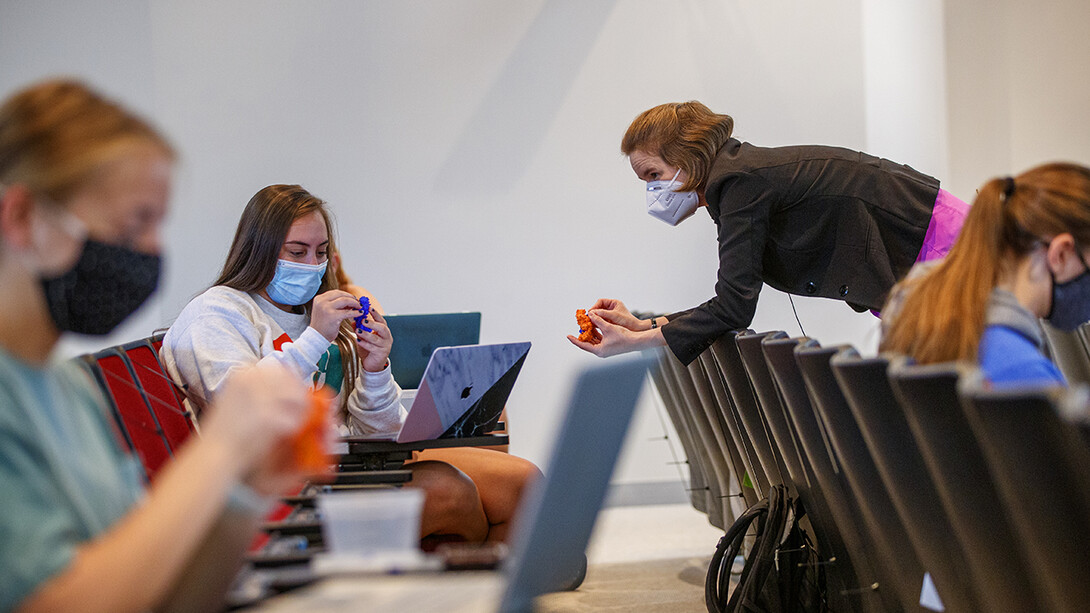
Biochemistry has a simple rule: Function follows form.
But those forms — the snarls of amino acid chains that fold over and branch from one another to construct cellular machinery known as proteins, even the famous double helix of the genetic instruction manual known as DNA — are anything but simple.
Just ask the University of Nebraska–Lincoln’s Rebecca Roston. During her postdoctoral appointment at Michigan State University, Roston researched under faculty she called “brilliant,” minds that had spent decades studying and teaching the boggling complexities of biochemistry. If anyone could mentally grasp those forms, which direct the behavior of the biomolecules essential to all life, it was them.
So when Roston presented her supervisors with some 3D-printed proteins, she was surprised to find that even they seemed to understand the structures better after interacting with the models.
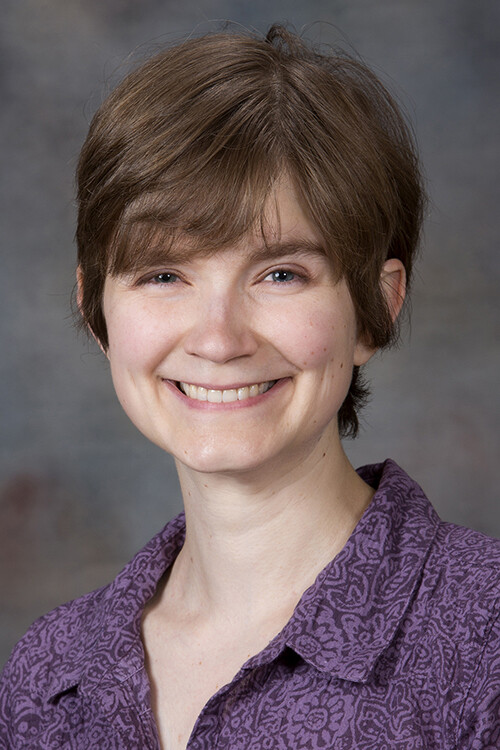
“And that really made something click for me,” said Roston, now an associate professor of biochemistry at Nebraska. “Conceptualizing the 3D structure of something is not just about straight-up intelligence. This is something that is really dependent on another set of skills that we don’t usually teach in biochemistry.”
Where many saw the problem, Roston and Karin van Dijk also saw an opportunity. For the past several years, the Husker biochemists and their colleagues have been busy researching the educational value of 3D-printed models, incorporating those models into biochemistry courses, and developing curricula and resources to help other interested faculty do the same.
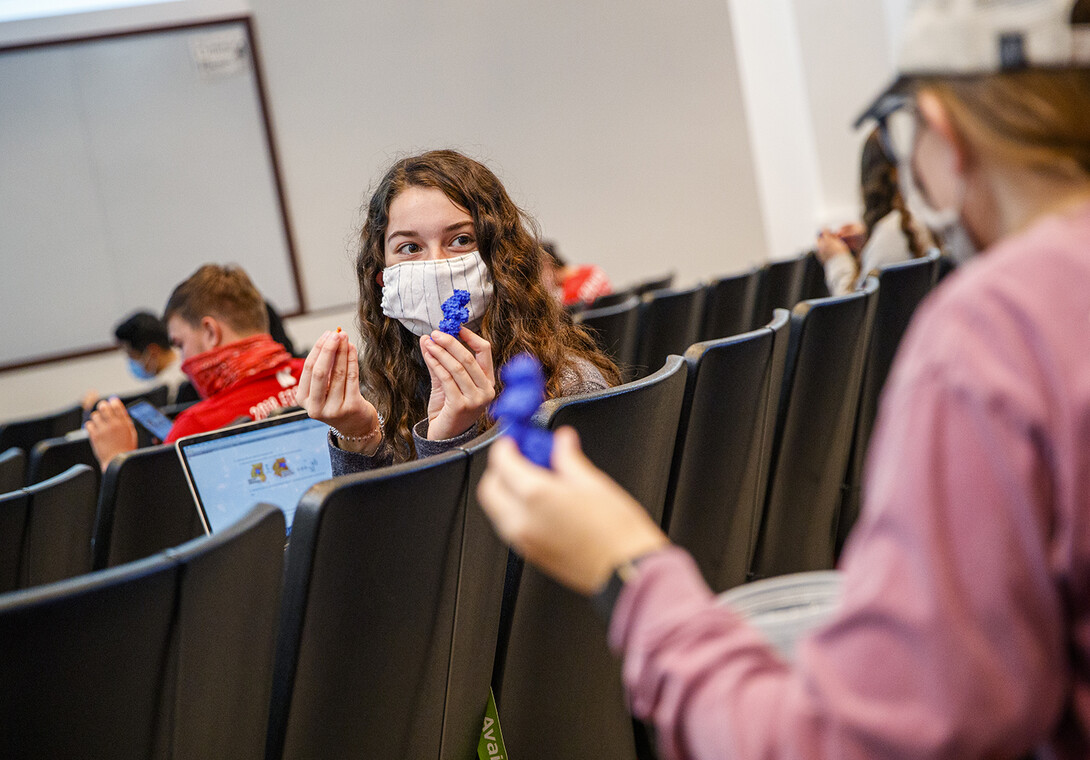
Their efforts ultimately aim at helping students better understand the nonstop flurry of life-sustaining, and occasionally life-threatening, activity playing out in every cell. Take proteins: A functional human cell houses tens of thousands of types, each with specific responsibilities that range from transporting nutrients and catalyzing chemical reactions to supporting structural membranes and transmitting signals to other cells. And each protein type folds into a configuration as unique as its responsibility.
Understanding the activity of a cell, then, means understanding the architecture and composition of its proteins, often down to the atomic or subatomic level. Those details also guide the design of antibiotics, inhibitors, antiviral drugs and other therapeutic agents that can help set right what goes wrong in a cell.
Unfortunately, trying to convey that information in two dimensions — or even three, using virtual renderings or the sorts of simplistic stick-and-ball models familiar to anyone who’s taken high school chemistry — can sometimes lead students astray, Roston and van Dijk said. The students can misperceive where and how molecules latch on to DNA. They can overestimate how much the joint of a biomolecule, where a bond connects two amino acids, can actually rotate. They can come to believe that the interior of a protein contains lots of open space.
“We all know that you’ve got to be able to see biochemistry,” van Dijk said. “I think that’s one of the most important things that students struggle with. They don’t (always) see how things dynamically change, how they behave based on their structure.”
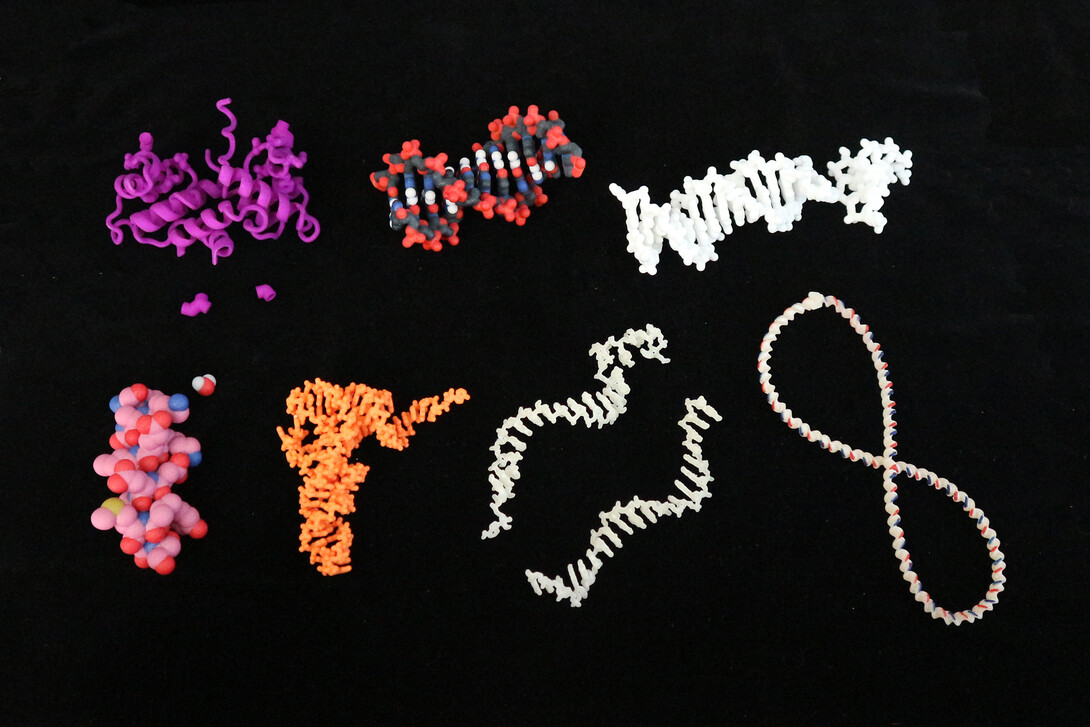
Roston and van Dijk recognized a potential solution in 3D-printed models. Those models could be printed from pliable materials, allowing for the twisting and shifting common in actual DNA, RNA and proteins, plus accommodate magnets to mimic the chemical bonds that hold biomolecules together.
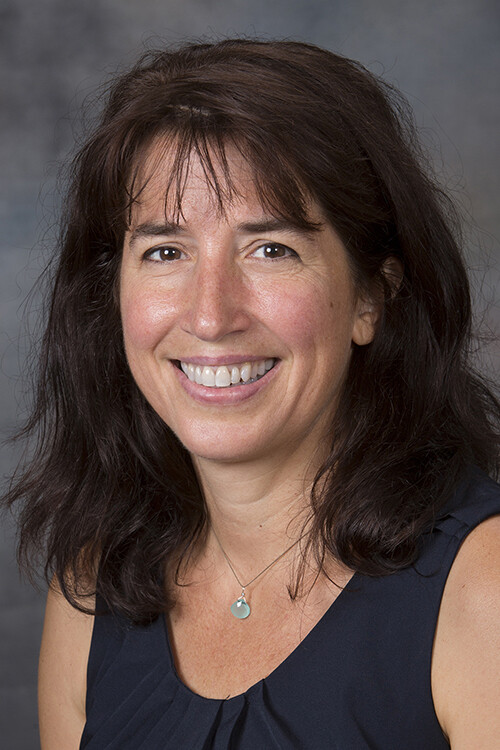
“We didn’t want to use only premade models,” said van Dijk, associate professor of biochemistry. “We actually wanted to try to do things that were somewhat dynamic. Instead of having these models that are 3D but just (static), we actually made models that move a little bit and change a little bit based on handling by students.”
Still, the researchers had good reason to suspect that maximizing the value of the models would require more than just dumping a bunch onto desks and asking students to start playing with them.
“There’s really good evidence from the chemistry field, where models are used a lot, that it’s not just the model — it’s how you use it with the students,” Roston said. “It’s not enough to just have a cool model set; you really have to have a way for the students to interact with it that will lead them to a certain outcome.
“Practically speaking, you can do a demo at the front of the classroom. That’s going to get some number of students — usually the good students. They’re already focused and paying attention. You can (also) request that they purchase a model set, like happens in a lot of chemistry classes, and then stick a couple in the library and assign homework that uses them. But again, if the students can Google the question and get the answer, they’re going to do that instead.”
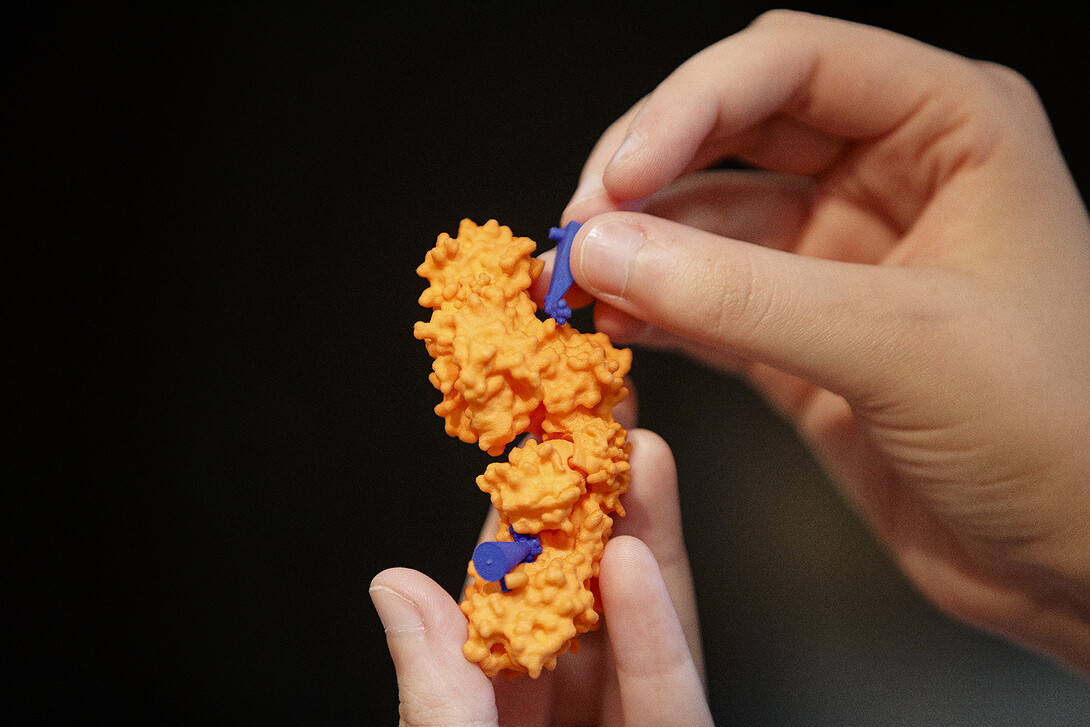
So Roston and van Dijk, alongside postdoctoral researchers Michelle Howell and Christine Booth, decided to build, test and refine learning modules for the two 400-level biochemistry courses in which they were introducing 3D-printed models. Each module would emphasize active participation and address some misconception or concept that prior research, combined with years of experience at the head of a classroom, had indicated was especially slippery.
One module addresses the way that DNA strands can coil or uncoil, which, among other effects, can restrict or give other biomolecules access to certain of its genes. Magnets within the model let students fasten or unfasten segments of the DNA strands to either tighten or loosen the coil, recreating the behavior of an enzyme that does it to help transcribe genetic blueprints or assist the DNA in replicating.
Throughout the module, which takes about 50 minutes of class time, students work together to answer questions in between snippets of lecture and demonstrations from their instructors. A web-based supplement offers immediate feedback on their answers and follows up with other questions according to how well the students are faring.
“We targeted educational goals that we had in our core biochemistry classes, because we thought that those would be the base level — the most applicable to the most people in the most places,” Roston said. “Almost everybody is using one of those big, fat biochem textbooks that goes through the material in a very similar way, so we target what’s in those.”
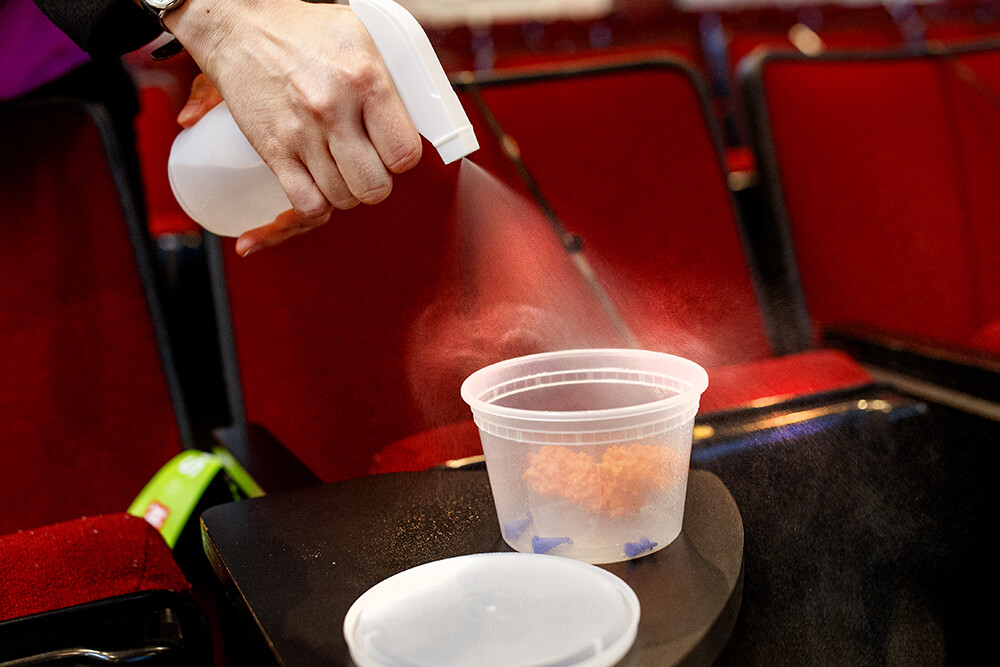
To test the effectiveness of the models and modules, the team turned to Brian Couch, associate professor of biological sciences. The researchers assessed student knowledge before and after the modules, comparing their knowledge gains against those of students who learned the same content without the modules. In one study, the team found that students who learned from the modules improved their understanding of biomolecule structure-function relationships by 49%, versus just 9% among the non-module group. Almost 60% of the module group also said that the models made the content easier to learn.
“(Using the 3D models) can only help you,” one student wrote. “To read something doesn’t really process, and then to hear it in lecture, you kind of get a feeling for it, but physically seeing it makes something abstract very real, and I feel like I got a lot more out of the physical modules.”
The models are finding their way into other Nebraska classrooms, too. The team tested its biochemistry modules in the classroom of Doane University’s Sharmin Sikich, where they seemed to be even more effective than in the larger University of Nebraska–Lincoln courses. Two postdoctoral researchers and a recent graduate of the University Honors program created basic biochemistry modules now in use across Lincoln Public Schools.
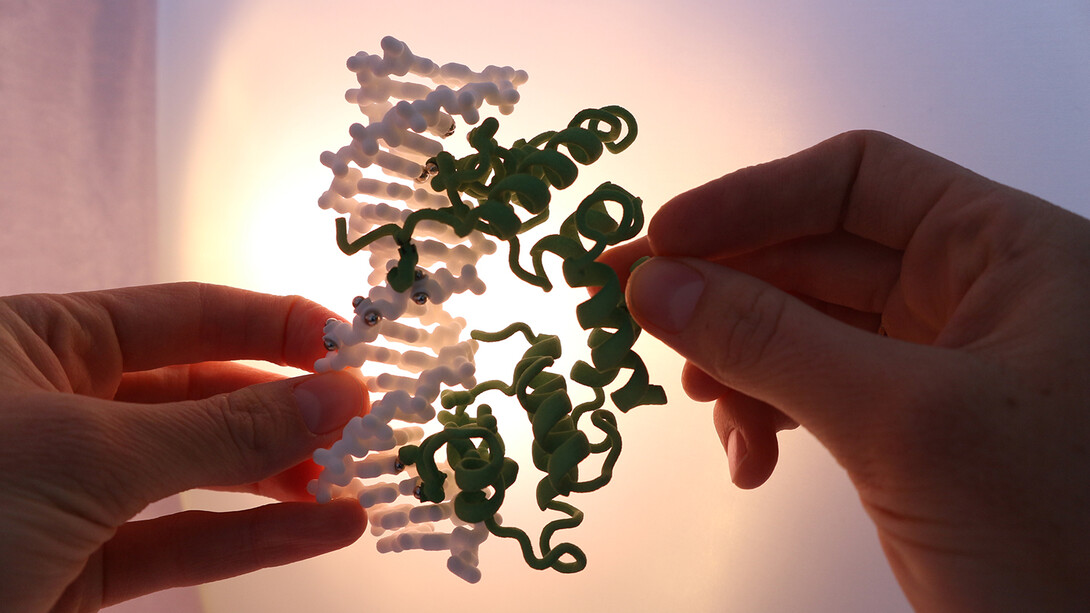
But the team is also promoting their benefits and use well beyond Nebraska, publishing guides on where to find detailed biomolecule blueprints, how to modify them using free 3D-rendering software, and how to export the files into formats readable by 3D printers. The falling costs and rising availability of those printers is making them ever-more accessible for faculty who are seeking ways to make biochemistry more accessible for their students, Roston said.
“A lot of places with decent engineering departments have really nice 3D printers, and they’re looking for projects for them,” she said. “For the people who have access to those, this is next to free.”