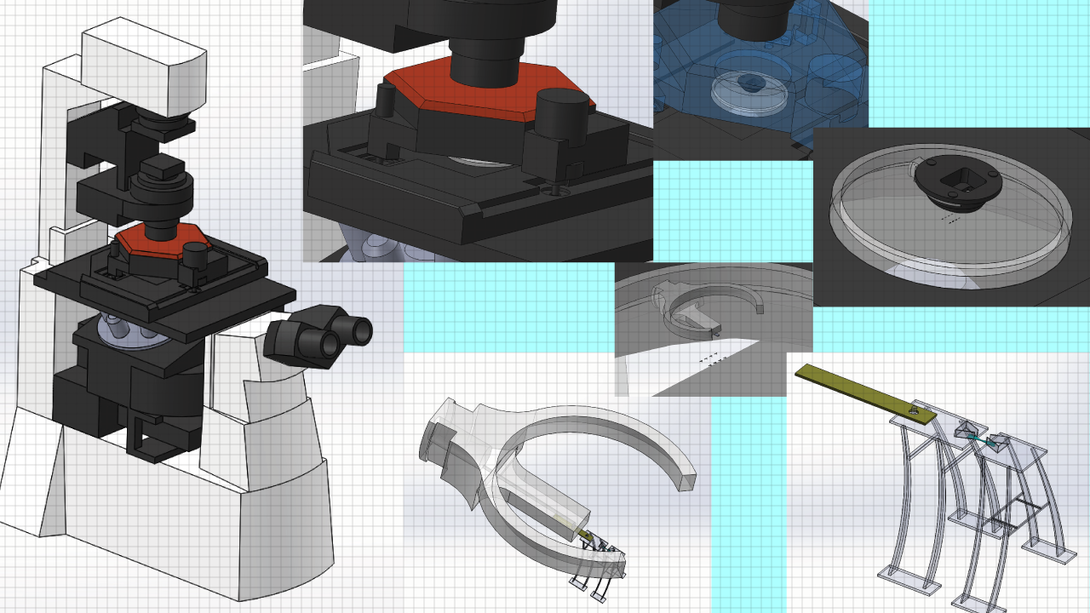
It took less than a day for the pair to form an organic connection that helped anchor them, that helped them communicate and share resources, that put them on the path to becoming part of something bigger than themselves.
It took the University of Nebraska–Lincoln’s Ruiguo Yang far longer to devise a way to put that connection to the test, to ratchet up the stress with unprecedented precision, all while measuring the strain it could endure before reaching a breaking point.
The pair in question? Human cells. Their connection? A physical junction that forms between them and, when joined by more friends, allows them to form tissues. Yang, an assistant professor of mechanical and materials engineering, has nothing against the cells he’s been so busy stressing out. On the contrary: The newly published work he’s spearheaded might ultimately help save untold numbers of them.
Thanks to a painstaking feat of microscopic engineering, Yang and his colleagues have now managed to record the biomechanical behavior of mature, individual cell-to-cell junctions for the first time, as reported in Proceedings of the National Academy of Sciences.
Though it spans only thousandths of a millimeter, a junction has outsized influence over the fates of the cells it connects. Depending on the type, it can take on many roles: a shepherd that keeps cells from drifting apart, a line of chemical communication between them, a gateway for the transport of water and nutrients.
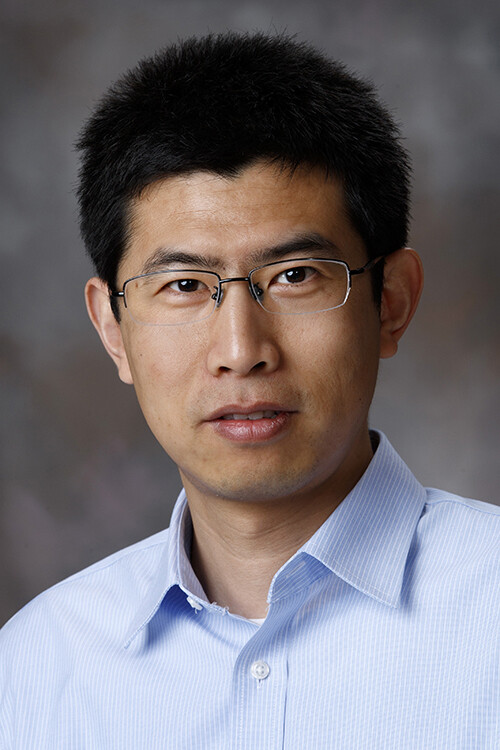
“Hopefully, that information will come in handy when it comes to understanding (human) development and disease.”
The team was especially interested in the junction’s stresses and strains — usually the macro-level concerns of construction and civil engineers. Stress describes the amount of force applied to a given area; strain describes how much an object stretches or otherwise deforms in response to that stress. The more stress an object can sustain without snapping, the greater its tensile strength.
Even when separating two cells at a relatively rapid rate, the researchers found that their junction could stretch to more than twice its original length before breaking.
“It’s remarkable that they can take that much strain before they rupture,” Yang said.
Still, the team was applying and measuring almost inconceivably tiny forces on the tiny scale of cells, which are often less than 1/50th of a millimeter in diameter. No one had ever figured out how to both control and record those forces with the precision needed to study the dynamics of a lone cellular junction in its natural, fully developed state. Earlier efforts were limited to testing junctions whose cells were in a suspended rather than an anchored state, making the results less generalizable and relevant to the actual goings-on in the body.
Yang’s solution to the longstanding challenges involved building two trapezoidal platforms that, when placed together, resemble a microscopic bowtie. Each platform is bordered by three-sided walls to keep a deposited cell from leaving the platform but enable it to make contact, and form a junction, with the cell across from it. The cells also anchor themselves to their platform bases in much the same way that they do in the body. Moving either platform away from the other, then, begins imposing stress on the junction between them.
But the design required another component, one that would pose a substantially greater engineering challenge. Beneath each platform would rest a series of support beams whose stiffness would approximate, as closely as possible, the stiffness of the cellular junction. By moving one platform away from the other, recording the deformation of the support beams beneath each platform, and then correlating the magnitudes of the two, the researchers could effectively calculate the stress and strain on the cellular junction.
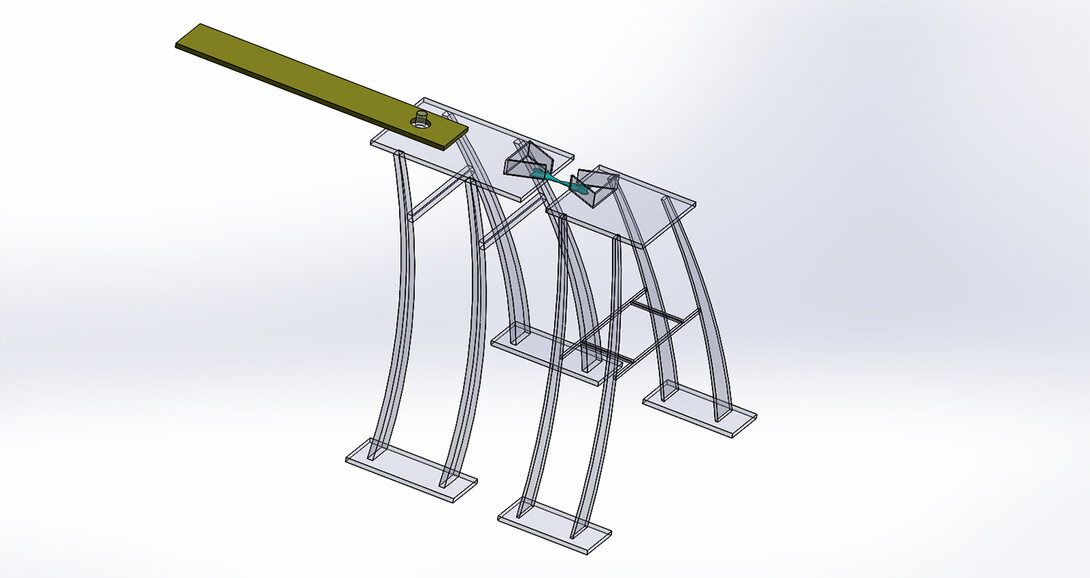
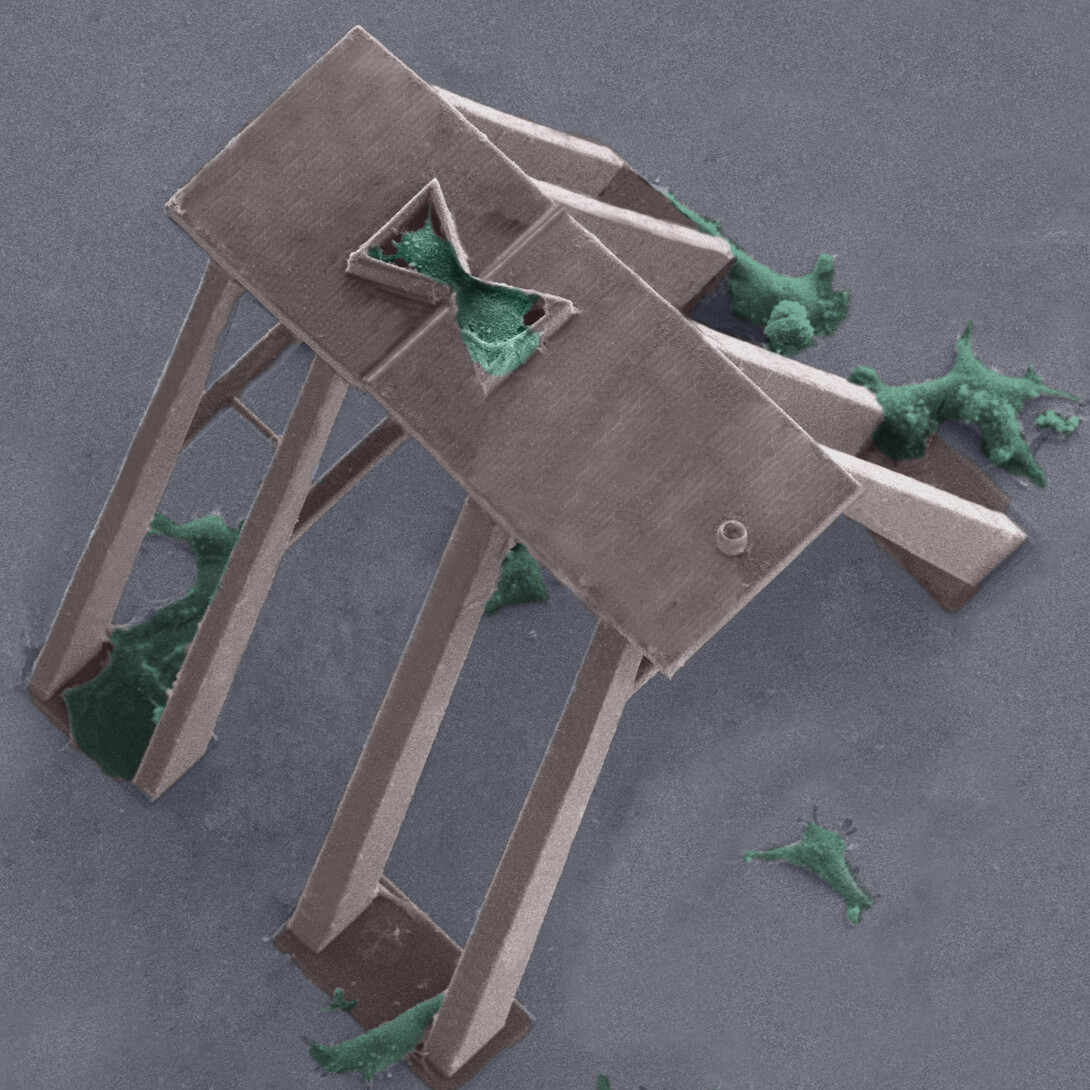
To meet the demands of size, complexity and sensitivity, the team decided to fabricate the beams from a soft polymer, using lasers to solidify bits of gel into the desired architecture. Yang and his colleagues first tried fabricating horizontal beams before finally conceding that they couldn’t achieve the needed flexibility. So they turned to vertical beams — which themselves tended to collapse until the team added microscopic trusses, creating A-shaped supports that finally did the trick.
“To balance the softness and the stiffness — the softness to detect the forces and the stiffness to stand up to the fabrication process — was a very, very big challenge,” Yang said. “It took more than a year to actually get to that point.”
After hitching their new apparatus to a so-called atomic force microscope, which can generate forces as small as a nano-newton — roughly 1 billionth the force of an apple falling from a tree — the team began subjecting the cellular junctions to various amounts of stress at various rates.
When the researchers rapidly tugged one of the platforms away, the junction seemed to bear the full brunt of the stress, snapping quickly in response. When they separated it at a lower rate, though, “the behavior was totally different,” Yang said. Rather than snapping under the same level of stress, the junction continued to stretch, exceeding its tensile strength in the other scenario.
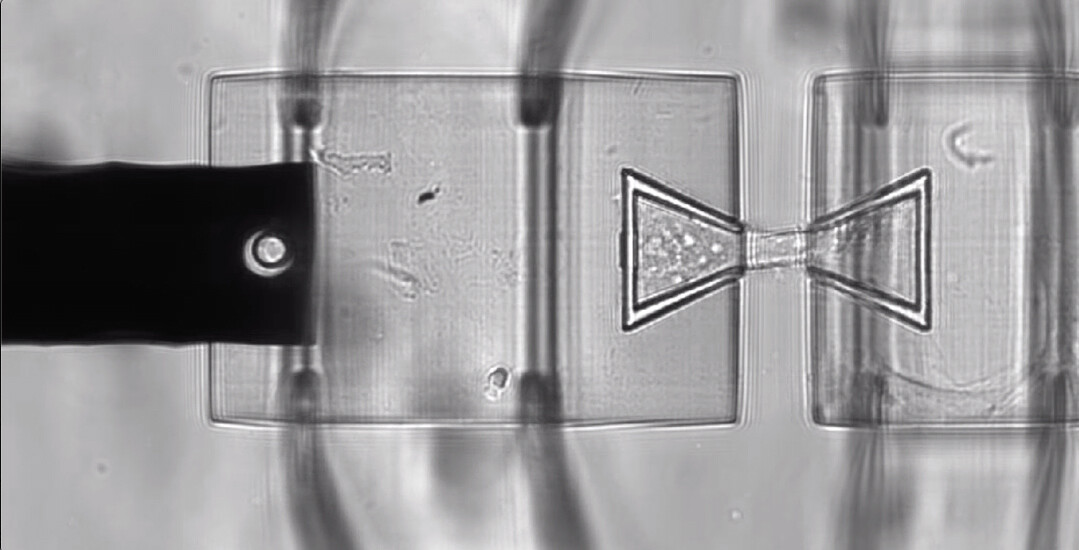
Why the difference? The team suspects it’s due mostly to a dynamic response not from the junction but from the cells themselves. Many types of cells, including the epithelial cells tested by Yang’s group, contain a cytoskeleton: essentially an interlacing network of molecular chains. True to its name, the cytoskeleton exists in large part to provide a cell with structure and support. Yet it can also remodel itself on the fly, reconfiguring those chains to contract the cell, for instance, or assist it in migrating. Yang thinks the cytoskeleton pulled a version of the same trick to dissipate some of the stress that his team imposed on the cells.
“They have some sort of internal sensor, most likely, so they sense that they are in danger of stress build-up,” he said. “If they can grow the chains longer, then the amount of stress within a cell will be lower. It’s like stretching a bungee cord or a rubber band. The rubber bands are actually growing longer. They grow and relax the tension, and the relaxed tension avoids rupture (of the junction).
“But if you pull them really quickly, they don’t have that time to adapt.”
That adaptation is a prime example of mechanotransduction: how mechanical stimuli can alter the behavior, and sometimes even the genetic makeup, of cells. The fact that suspended cells don’t exhibit those adaptations, Yang said, highlights the importance of studying their behavior in their mature, anchored state.
The team’s discoveries could rate as the first of many to be gleaned from using its system, Yang said. Some of those discoveries might eventually inform the development of therapeutic treatments designed to prevent the junction failures associated with some heart diseases and other maladies.
“In the current testing of drugs and therapeutics, people rely on (testing) a monolayer of cells,” he said. “You dump them in the media and see the mass effects of it. But we are looking at one junction only to see precisely what is happening. Our goal is to provide the most basic, fundamental understanding, which can then be applied to other, more complicated conditions, where you have three-dimensional cell structures.”
The team demonstrated that potential by using its system to investigate the behavior of E-cadherin, a protein vital in forming and maintaining cell-to-cell junctions. Prior research has suggested that a deficiency of E-cadherin, which can lead to junction detachment and disrupt chemical signaling between cells, might contribute to the spread of cancerous tumors.
As they subjected a junction to strain at a very low rate, the researchers observed E-cadherins clustering around the junction. The clustering appeared to surge as the strain increased from 10% to 100% over the course of about 10 minutes, indicating that the E-cadherin behavior might also be contributing to the greater tensile strength observed under that condition — another potential hint of mechanotransduction at work.
“Although this data is very preliminary, there are many, many other datasets we’re currently working on,” Yang said. “But even the preliminary data can show us that this molecule may somehow regulate the mechanics of this junction, and also potentially regulate how the cells respond to the strain.
“If we can identify the functions of those junction molecules when they are being subjected to strain, then we can test the efficacies of certain drugs that might alter their fate. So we believe this potentially can be a drug-screening platform down the road.”
Yang authored the PNAS study with Nebraska’s Amir Monemian Esfahani, Jordan Rosenbohm, Bahareh Tajvidi Safa, Grayson Minnick, Xiaowei Jin, Eunju Kim, Ying Liu, Yongfeng Lu and Jung Yul Lim; the University of Nebraska Medical Center’s James Wahl III; Ming Dao of the Massachusetts Institute of Technology; Nickolay Lavrik of the Oak Ridge National Laboratory; and Quan Zhou, Fang Kong and Changjin Huang of Nanyang Technological University.
The researchers received support in part from the National Science Foundation and from the National Institute of General Medical Sciences, part of the National Institutes of Health, through the Nebraska Center for Integrated Biomolecular Communication.